Big picture: human pluripotent stem cells as a model for the embryo
How an amorphous lump of identical cells is able to develop into a human being is a profound question of equal intellectual and practical importance. Its fascination springs in part from the desire to know where we come from, and how we differ from other animals. Yet the ability to direct differentiation of cultured cells into distinct cell types for therapeutic use, perhaps even coax them to form complete organs, relies on answering the same question. Ethical considerations make experimentation on human embryos unacceptable and on other mammals undesirable. Moreover, significant interspecies differences complicate inference about human development from animal models. This challenges us to find answers in alternative ways.
Remarkably, human pluripotent stem cells (hPSC) retain the embryo’s innate capacity to self-organize. The embryonic body plan is laid out during gastrulation, when pluripotent cells commit to one of three different lineages -ectoderm, mesoderm or endoderm- that organize into three germ layers. Upon treatment with the differentiation-inducing molecule BMP4, hPSCs confined to a disc by micropatterned substrate mimic this process and reproducibly differentiate into concentric rings corresponding to each germ layer. Therefore, these micropatterned colnies are now commonly referred to as 2D human gastruloids. Importantly, the same genetic circuitry as in the embryo is orchestrating the pattern in 2D gastruloids. Therefore, by systematically varying the environment we can probe underlying mechanisms with throughput and precision unachievable in living embryos.
Read more about stem cells as a model for embryonic patterning, or as a platform to study early human development, in one of our recent reviews.
Research themes
Live imaging of signaling in hPSCs: fluorescent signal transducer moves into nucleus in response to morphogen
Morphogen signaling dynamics
Morphogens are diffusible signaling molecules thought to determine cell fates in a level-dependent way during developmental patterning. However, in the vertebrate embryo, cells experience changing morphogen levels during differentiation, making this static model insufficient. As one way to address this, we developed single cell tracking of signaling linked to cell fate and in a first application used this to determine how cells interpret BMP signaling. We discovered that time-integrated (total) BMP signaling controls differentiation: both the level and duration of signaling activity impact cell fate choices only by changing the time integral of signaling and the duration and level are therefore interchangeable in this context. In a stem cell model for embryonic patterning, we showed that that the integral model correctly predicts changes in cell fate domains when signaling is perturbed. Using a screen, we found 3 families of transcription factors including SOX may be involved in integrating BMP signaling. Direct manipulation of SOX2, live-cell imaging of SOX2 dynamics, genomic data, and mathematical modeling support a key role for SOX2 in integration of BMP signaling. We can now address follow-up questions such as 1) What are the molecular mechanisms by which SOX2 and other integrator genes are regulated by BMP? 2) Does integrated BMP signaling control differentiation beyond the first cell fate decisions we studied? 3) What happens when multiple cell signaling pathways combine? Read our paper on time-integrated BMP signaling.
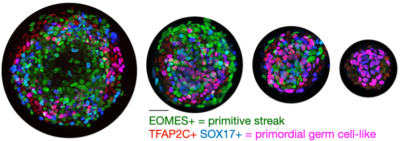
Human germ cell differentiation
Primordial germ cell (PGC) specification is the first step in the development of the germ line. Many aspects of human PGC specification remain poorly understood because of the inaccessibility of the early post-implantation human embryo for study. Filling this gap is of great value for infertility research and treatment, for example supporting efforts toward in vitro gametogenesis. We discovered that in 2D human gastruloids, PGC-like cells are reproducibly specified, and that on small micropatterns the fraction of PGC-like cells is consistently 70% compared to an average 30% by previous methods. Establishing a powerful experimental platform for hPGC-like differentiation enabled us to substantially improve our understanding of the signaling requirements for hPGC-like cells. Most importantly, we showed that Wnt –previously considered the key signal in controlling the decision between mesoderm and PGCs– acts indirectly to induce expression of Nodal, and that Nodal is the limiting factor in PGC-like specification. We developed a mathematical model for how relative timing and magnitude of BMP and Nodal controls the decision between amnion, primordial germ cells, and primitive streak (~future mesoderm) that explains our experimental data. This model implies a two-dimensional state space in which different trajectories can be followed, passing through a more amnion-like or mesoderm-like intermediate state depending on whether cells are first exposed to BMP or to Nodal. We believe this may resolve a debate in the field about whether PGC progenitors are more amnion-like or mesoderm-like: it can be both. We are now building this work to understand how maintenance of germ cells is controlled by different cell signals than induction. Read our paper on micropatterned differentiation of PGC-like cells and our perspective on PGC differentiation.
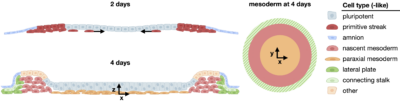
Human mesoderm development
Due to their simplicity and reproducibility, 2D gastruloids are ideal for high throughput quantitative studies of tissue patterning and have led to many insights into the mechanisms of mammalian gastrulation. However, until recently they were only studied for 2 days. My lab extended this system to 4 days and discovered a phase of highly reproducible morphogenesis during which directed migration from the primitive streak-like region gives rise to a mesodermal layer beneath an epiblast-like layer. Multiple types of mesoderm arise with striking spatial organization including lateral mesoderm-like cells on the colony border and paraxial mesoderm-like further inside the colony. Single cell transcriptomics showed strong similarity of these cells to mesoderm in human and non-human primate embryos. This illustrates that extended culture of 2D gastruloids provides a powerful model for human mesoderm differentiation and morphogenesis. Having established the first model of human mesoderm migration with this work, we can now leverage it to dissect the mechanisms underlying mesoderm migration, an essential developmental process about which little is known. Check out our preprint on mesoderm patterning in human gastruloids.
Role of tissue geometry and topology in patterning
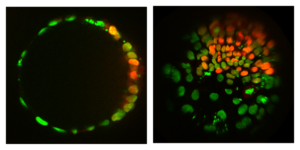
Understanding the interplay between pattern and shape, through which the body plan is established, is a major challenge in developmental biology. Unlike in embryos, geometry (shape) and topology (how things are connected) of stem cell colonies can be controlled to study the effect on patterning. The simplest models for spatial differentiation in micropatterned stem cell colonies depend on the existence of a colony border. However, by growing cells on spheres we found that patterning still occurs. This suggests that the underlying mechanism is the spontaneous formation of a morphogen gradient through an activator-inhibitor system, as suggested long ago by Alan Turing. Looking at patterning on surfaces with different symmetry and shape will further constrain the mechanism of symmetry breaking and provide insight into the establishment of the body axes.